Developments in the pharmacotherapeutic management of spontaneous preterm labor
KY Ronald Kam & Ronald F Lamont†
†Imperial College London, Department of Obstetrics and Gynaecology, Northwick Park and St Mark’s NHS Trust, Watford Road, Harrow, Middlesex HA1 3UJ, UK
Background: Preterm birth is the major cause of perinatal mortality and morbidity in the developed world. Objective: The aim of this study was to establish the importance of preterm birth and the huge healthcare costs involved and review the pathophysiology of preterm labor and the use of antepartum glucocorticoids, which are the main reason why tocolytics are used to prevent or delay preterm birth. The study also reviewed the range of tocolytics available, their mode of action and the evidence for their efficacy and fetomaternal safety.
Methods: An extensive review of the literature using well-recognized and accepted scientific search engines was employed. Results/conclusions: The perfect tocolytic does not exist. The evidence to support the use of magnesium sulfate as a tocolytic is poor. The use of β-agonists is decreasing worldwide as clinicians move to nifedipine or atosiban, which are as effective but much safer. Although nifedipine is cheaper than atosiban and can be administered orally, the evidence to support atosiban is much superior to that of nifedipine and there have been recent safety concerns over nifedipine.
Keywords: antagonists, atosiban, β-agonists, labor, nifedipine, oxytocin, premature, preterm, receptor, tocolytics, vasopressin
Expert Opin. Pharmacother. (2008) 9(7):1153-1168
1. Importance of preterm birth
Preterm birth is defined as birth before 37 completed weeks of gestation. Approximately 13 million preterm births occur worldwide annually, ranging from an incidence of 11% in North America to 5.6% in Oceania. Europe, with an incidence of 5.8%, accounts for 400,000 preterm births annually, 100,000 of which are thought to be potentially preventable [1]. Preterm birth is the major cause of perinatal mortality and morbidity in the developed world. At 24 weeks of gestation 80% of babies die, yet by 30 weeks of gestation 90% of babies survive and by 40 weeks of gestation over 99% of babies survive [2]. This means that it takes 10 weeks (30 – 40 weeks of gestation) for survival to increase by approximately 10%. In contrast, between 22 and 26 weeks of gestation a few days of delay may be sufficient to improve survival by 10% [3]. While 50% of preterm births occur after 35 completed weeks of gestation, nearly all of the mortality occurs before this time [2]. In a study of the morbidity associated with preterm birth, the EPICURE Study reported on the outcomes of babies born between 22 and 26 completed weeks of gestation. Approximately 65% of such babies died in the delivery suite or in the neonatal intensive care unit (NICU). Of those who survived to be discharged home, at 30-months follow-up approximately 50% had some form of handicap and in 50% of these the handicap was severe [4].
The cost of neonatal intensive care in the US is $10,000 per baby per week and approximately $5 billion annually. If a child is handicapped and requires lifelong residential care, the cost is estimated to be $450,000 [5]. In the UK, when excluding NICU costs, which are approximately £800 per day, the cost of hospital readmissions over the first 5 or 10 years of life for babies born before 28 completed weeks of gestation is 20 times greater than for babies born after 37 completed weeks of gestation [6]. The intangible costs of preterm birth due to loss of earnings and the travel expenses of the parents and extended family are difficult to calculate and the psychosocial costs of preterm birth are incalculable. Approximately one-third of all preterm births are elective or iatrogenic for fetomaternal indications. Another one-third of preterm births occur following preterm prelabor rupture of the membranes in which tocolytic drugs are relatively contraindicated and the remaining one-third are due to spontaneous preterm labor (SPTL) with intact membranes and so may be suitable for tocolytic therapy. Recently, the Preterm Labor Working Party of the European Association of Perinatal Medicine published guidelines on the management of SPTL, which include the diagnosis of SPTL, the relative and absolute contraindications to intervention and the use of tocolytics [7].
2. Pathophysiology of preterm labor
The final common end pathway of human parturition is a complex and poorly understood event that involves different endocrine,paracrineandbiochemicalmechanisms.Theseact to convert the uterine environment from one of relative quiescence to a dynamic state characterized by increasingly frequent, longer, stronger and more synchronous uterine contractions, together with substantive changes in the uterine cervix leading to effacement and dilatation. The factors that trigger parturition in primates and humans are unknown. Duringpregnancy, progesteroneisresponsibleformaintaining uterine quiescence [8]. In humans, the maternal serum levels of progesterone and estrogen do not change significantly around the time of labor so, unlike many mammalian species, the onset of labor cannot be attributed to progesterone withdrawal [9]. The parturition-inducing effect of progesterone withdrawal appears to be achieved through functional resistance of the myometrium to the effects of progesterone. There are two major subtypes of progesterone receptor: PR-A and PR-B. The latter subtype mediates responsiveness to progesterone while the former has a suppressive effect [10]. Functional progesterone withdrawal is thought to result from a relative increase in PR-A compared to PR-B [11]. Despite high circulating levels, estrogen, which can promote myometrial contractility by upregulating the expression of genes such as the oxytocin receptor, has little effect on the myometrium for most of the duration of normal pregnancy [12]. Functional progesterone withdrawal removes the inhibitory effect of progesterone on the expression of estrogen receptor α (ERα) and the subsequent increase in ERα expression allows circulating estrogen to induce uterine contractility, a process known as functional estrogen activation [13].
The biochemistry of labor closely resembles that of inflammation, with upregulation of prostaglandins (PGs) and pro-inflammatory cytokines [14]. Functional progesterone withdrawal may also result from activity of the nuclear factor kappa B (NF-κB) family of transcription factors. NF-κB and progesterone receptors reciprocally suppress each other in the amnion and may do so in other tissues such as in the mammary gland, ovaries and endometrium [15,16], where progesterone- and NFκB-regulating cytokine receptors are expressed together. NF-κB activity is intimately linked to pro-inflammatory and pro-labor effects. NF-κB exerts its pro-labor effects by upregulating the expression of both COX-2, which increases PG production and IL-8, which act together to cause increased uterine activity and cervical compliance [15]. NF-κB is a key component underlying inflammation, regulating the expression of many pro-inflammatory genes and the production of cytokines, immune receptors, enzymes and other pro-inflammatory molecules [17]. The NF-κB levels increase at the onset of labor and may promote those cases of labor that are due to infection [11,18].
During labor, uterine contractions are coordinated by the presence of gap junctions between myocytes, which allow action potentials to move between and trigger the contraction of adjacent cells so that the myometrium functions as a syncytium [14]. Intracellular calcium levels govern smooth muscle tone through the binding of calcium to calmodulin to form calcium–calmodulin complexes. These complexes activate the enzyme myosin light chain kinase, which in turn phosphorylates myosin, enabling myosin heads to interact with actin filaments, which allows cross-bridge formation and muscle contraction [9]. Intracellular calcium concentrations increase when calcium ions enter the myocyte via voltage-gated calcium channels in the cell membrane or when calcium is released from stores in the sarcoplasmic reticulum. The mechanisms by which uterine contractility appears to be modulated generally involve a change in the intracellular calcium concentration brought about by enzyme pathways under the control of G protein-coupled receptors. Uterine contractility can be triggered by coupling between the oxytocin receptor and Gq/11 proteins, which results in phospholipase C β-mediated hydrolysis of phosphatidylinositol-4,5-bisphosphate, producing inositol-1,4,5-trisphosphate (IP3) which increases calcium release from the sarcoplasmic reticulum [9]. Conversely, uterine relaxation can be achieved through binding to certain G protein-coupled receptors (e.g. β2-adrenoceptors) coupled to Gαs proteins, resulting in increased adenylyl cyclase activity and, hence, cAMP formation, which facilitates protein kinase A [9]. Protein kinase A is an enzyme that mediates phosphorylation of specific cellular substrates, but how its activity promotes uterine relaxation remains unclear. Phosphodiesterase 4 is an enzyme that is responsible for the degradation of cAMP in specific cell types [19] and its inhibition by progesterone in the uterus constitutes further evidence of the role of cAMP in mediating uterine quiescence [9,20].
3. Antepartum glucocorticoids in the management of preterm labor
The effectiveness of antepartum glucocorticoids administered to mothers at risk of preterm birth in order to accelerate fetal lung maturity is widely accepted and antepartum glucocorticoids should be given to women at risk of preterm birth between 24 and 34 weeks of gestation [21,22]. Lack of surfactant caused by immaturity of lung development results in hyaline membrane disease and neonatal respiratory distress syndrome (RDS). This accounts for most of the neonatal morbidity and mortality associated with preterm birth. Respiratory distress syndrome results from quantitative and qualitative deficiency of lung surfactant and also from an insufficient number of alveoli capable of efficient gaseous exchange. Lambs delivered preterm after maternal infusions of corticosteroids survived and possessed lungs that demonstrated accelerated lung maturation. Consistent with this, Liggins and Howie [23] found in the first randomized controlled trial (RCT) of antepartum glucocorticoid therapy that the risk of RDS was significantly reduced in the treatment group compared to placebo. This effect has since been confirmed by a large number of RCTs, which have recently been comprehensively reviewed [24]. Treatment with antepartum glucocorticoids significantly reduces the risk of RDS by 34% (relative risk (RR) = 0.66 and 95% CI = 0.59 – 0.73) and also reduces the risk of neonatal death (RR = 0.69 and 95% CI = 0.58 – 0.81). Glucocorticoid treatment also reduces the incidence of other neonatal complications of preterm birth such as necrotizing enterocolitis (RR = 0.46 and 95% CI = 0.29 – 0.74), intraventricular hemorrhage (RR = 0.54 and 95% CI = 0.43 – 0.69) and systemic infection within the first 2 days of birth (RR = 0.56 and 95% CI = 0.38 – 0.85). There is a reduced need for admission to the NICU in those treated with antepartum glucocorticoids (RR = 0.80 and 95% CI = 0.65 – 0.99) and treated babies require less neonatal respiratory support.
3.1 Biological actions of antepartum glucocorticoids on the fetus
Administration of antepartum glucocorticoids accelerates fetal lung maturation, which improves gaseous exchange and lung compliance. In fetal sheep there is a reduction in the lung mesenchymal thickness and decreased alveolar septation [25]. While this resembles severe bronchopulmonary dysplasia [26], the effect appears to be reversible and does not affect lung function at term [27]. Corticosteroids regulate the production of both the lipid and protein components of fetal lung surfactant [28,29]. The production of surfactant appears to increase after a delay of 4 – 7 days following antepartum glucocorticoid administration [30] and the levels of mRNA for surfactant proteins increase only transiently after therapy before returning to basal levels despite repeated doses of betamethasone [29].
In the most recent large review of antepartum glucocorticoid therapy, birth-weight was not significantly lower in babies exposed to a single course of antepartum glucocorticoids [24]. In contrast, intrauterine growth restriction has been reported in human fetuses subjected to repeat courses of antepartum glucocorticoids [31]. Corticosteroids have also been shown to accelerate maturation of other fetal tissues, including the intestine, liver, pancreas, kidney, adrenal, skin and myocardium [28]. The accelerated maturity of the fetal intestine may underlie the reduced risk of necrotizing enterocolitis associated with antepartum glucocorticoid therapy [24,28]. Antepartum glucocorticoids also appear to cause vasoconstrictive effects in the fetal cerebral vasculature, which may protect the fetus from intraventricular hemorrhage [32].
3.2 Current controversies in the use of antepartum glucocorticoids
3.2.1 Long-term adverse effects
Increased corticosteroid exposure during fetal development may result in low birth-weight and unhealthy adult outcomes hypothesized as part of the ‘fetal origins of adult disease hypothesis’ [24,33]. Disorders of health in childhood suspected to have their origins in antepartum glucocorticoid therapy include hypertension, insulin resistance and diabetes and poor psychological and cognitive function. Recently, 30- and 31-year follow-up studies on the patients involved in the first RCT in New Zealand [23] have been reported [34-36]. The results were reassuring. No differences were found between the treatment and placebo groups with respect to lung function and the prevalence of asthma or other respiratory disease [34]. In addition, there were no clinical effects of antepartum glucocorticoids on body size or cardiovascular risk factors such as hypertension, an abnormal lipid profile, prevalence of diabetes or history of cardiovascular disease, but those exposed to antepartum glucocorticoids had greater insulin resistance on glucose tolerance testing, a finding of uncertain clinical significance [36]. Cognitive function, working memory and attention, psychiatric morbidity, handedness and health-related quality of life did not differ significantly at 31 years of age between antepartum glucocorticoid and placebo groups [35]. In summary, no long-term adverse outcomes were identified which would justify withholding the use of antepartum glucocorticoids in pregnancies at risk of preterm birth.
3.2.2 Single or repeat doses
A reduction in neonatal mortality is seen in treated infants born within 48 h of administration of a single dose of antepartum glucocorticoids but not in infants born 7 days after administration [24,37]. Despite the lack of a clear evidence base, this observation led to the introduction of repeat courses of antepartum glucocorticoids to women who remained undelivered within 7 days of the first administration but who were still at risk of preterm birth [38]. In a meta-analysis of three RCTs of 551 women, repeat courses of antepartum glucocorticoids appeared to reduce the severity of neonatal lung disease and the need for surfactant administration but not perinatal mortality when compared to a single administration [31]. A recent RCT involving 982 women showed a significant reduction in the incidence of RDS and severe lung disease in babies exposed to repeat courses, but failed to examine for a reduction in neonatal mortality [39]. In common with other studies in which repeat courses were employed, small decreases in birth-weight and head circum- ference were observed [40-43]. Neonatal adrenal suppression due to repeated antepartum glucocorticoid administration has also been demonstrated, giving rise to fears of an inadequate neonatal cortisol response to stress [41,44,45]. Due to possible long-term adverse effects of repeat antepartum glucocorticoid administration, the routine use of repeat courses of antepartum glucocorticoids should be discouraged and single courses should be optimized [46].
4. Tocolytic agents
Tocolytics are used to inhibit or stop uterine contractions in order to delay delivery in women in SPTL with intact membranes prior to 34 weeks of gestation. There are three main indications for the use of tocolytics: firstly, for delay of delivery so that a course of antepartum glucocorticoids may be administered, secondly, to allow time to arrange in utero transfer to a centre with NICU facilities and, thirdly, to delay delivery in order to allow further maturation and growth for a putative increase in survival and decrease in handicap.BeforepharmacotherapyforthetreatmentofSPTL wasintroduced,maternalbedrest,sedationoranalgesiawere employed. Maternal hydration was also used, the scientific basis for which has only subsequently been elucidated. The myometrium contains not only oxytocin receptors but also vasopressin receptors of type V1a (as opposed to V1b or V2 present in other tissues), the stimulation of which results in uterine contractions. Maternal hydration promotes a diuresis by causing a reduction in vasopressin (antidiuretic hormone) secretion with less stimulation of V1a and oxytocin receptors, for which there is crossover affinity. Alcohol also inhibits posterior pituitary secretion of oxytocin and vasopressin (leading to diuresis and dehydration) and was also used to treat SPTL well into the 1980s, but had unacceptable side effects for women. In 1982 the US FDA permitted the introduction of ritodrine (Yutopar, Duphar) for the treatment of SPTL [47]. This more specific β2-adrenergic agonist replaced the use of the β1-agonist isoxsuprine (Duvadilin, Duphar), which, although developed for the treatment of peripheral vascular disease, was found to inhibit contractions. Over the next decade, β2-agonists such as ritodrine, salbutamol, terbutaline and fenoterol became established as the first-line therapy for the treatment of SPTL worldwide [48].
In 1992 the Canadian Preterm Labor Investigators [49] reported their findings and, in the same issue of the New England Journal of Medicine, an editorial by Leveno and Cunningham [50] called for a reappraisal of the use of β-agonists. This, together with the increasing concerns about the potentially serious side effects of β-agonists [51,52], led to a reduction in the use of β-agonists worldwide but mainly in the US. With no tocolytic alterative (atosiban was not registered for use in Europe until 1999), a number of tocolytic myths arose, which limited their use and/or resulted in a drift towards the use of other tocolytic agents with a poor evidence base. These are covered separately in another review [53]. A number of tocolytic agents are currently in use for the treatment of SPTL with differing strengths of evidence base for their safety and efficacy. Some are licensed for use (β-agonists and vasopressin/oxytocin (VOT) receptor antagonists), but most are not (nitric oxide donors, prostaglandin synthetase inhibitors (PGSIs), magnesium sulfate and calcium channel blockers (CCBs)). Although many drugs in use in obstetrics and neonatology are used off label [54], only one group was developed specifically to treat SPTL (VOT receptor antagonists) and the remainder (nitric oxide donors, PGSIs, CCBs, β-agonists and magnesium sulfate) were developed and introduced for other reasons, but were found to have possible tocolytic effects. Since they are not utero-specific they have multi-organ fetomaternal side effects. Each of these groups of agents will now be considered with respect to their mechanism of action (see Section 2) and to the evidence base for their fetomaternal safety and efficacy. Nitric oxide donors are rarely used, magnesium sulfate has little evidence to support its use as a tocolytic and β-agonists are slowly being replaced by either the CCBs, nifedipine or the VOT receptor antagonist atosiban. Accordingly, since this review addresses the recent developments in the pharmacotherapeutic management of SPTL, the remaining sections will concentrate on the recent controversies in the use of β-agonists, the CCB nifedipine and the VOT receptor antagonist atosiban.
4.1 b-agonists
4.1.1 Mechanism of action
Ritodrine is the most commonly used β-agonist in the treatment of SPTL and is a β2-adrenergic agonist that inhibits spontaneous or induced myometrial contractions [55]. β-agonists bind to G protein-coupled receptors, resulting in activation of adenylyl cyclase to form cAMP from ATP. The pathway by which cAMP induces myometrial relaxation remains uncertain.
4.1.2 Efficacy
Since 1966 a number of randomized, placebo-controlled trials using β-agonists for treating SPTL have been performed. A systematic review of a selection of these trials found that β-agonists reduced the number of women in SPTL who delivered within 48 h of treatment [56] and this correlated with an earlier systematic review [57]. In the largest RCT of ritodrine therapy to date, the Canadian Preterm Labor Investigators Group [49] assigned 708 women in SPTL to an intravenous infusion of ritodrine (n = 352) or placebo (n = 356). They found that, although ritodrine therapy could prolong labor, it did not significantly reduce perinatal mortality, increase the number of fetuses reaching term or have any beneficial effect on birth-weight in the treatment group compared to placebo. The investigators found an increase in maternal adverse effects such as chest pain and arrhythmias.
β-agonists have also been used orally for maintenance therapy after successful intravenous tocolytic therapy to attempt to prolong gestation and reduce the risk of recurrence of SPTL. While early placebo-controlled trials suggested that recurrent SPTL could be successfully avoided with oral maintenance therapy [58,59], later trials and a recent systematic review found no benefit in perinatal outcome and no reduction in the rate of recurrent SPTL [60-63].
4.1.3 Safety
4.1.3.1 Maternal effects
β-adrenergic receptors are widely distributed in extra-uterine tissues, particularly the cardiovascular system, which results in peripheral vasodilatation, decreased peripheral vascular resistance and reflex tachycardia to compensate for systemic hypotension. This cardiovascular stimulation frequently causes symptoms such as tachycardia, headache, palpitations, sweating, tremor and dyspnoea and there is an increased risk of arrhythmias, angina and myocardial ischemia in susceptible women [48].
The significant adverse effects of β-agonist administration are either cardiovascular or metabolic. β-agonists have the potential to cause life-threatening pulmonary edema through a number of pathophysiological factors. Iatrogenic fluid overloadortheuseofsmallervolumesofhyperosmoticfluids may precipitate pulmonary edema. The normal physiological adaptations to pregnancy result in plasma expansion. Due to changes in the renin–angiotensin–aldosterone system, both physiologically and drug induced [51], retention of sodium and water occurs. While there have been many reports of pulmonary edema occurring in women who received β-agonists, with published figures varying between 0.01 and 9% of women [50,51,64], the figure is probably around 0.5% [51]. The tachycardia associated with β-agonist therapy often results in a very short cardiac cycle in which there is insufficient time for diastolic ventricular filling, causing pulmonary venous congestion, which increases the risk of pulmonary edema. Finally, up to 40% of cases of SPTL are due to infection [65] and the resultant tissue permeability predisposes to pulmonary edema. Women with pulmonary edema caused by β-agonist therapy usually respond to treatment but over 30 deaths have been reported [66-69] and, in 1989, 5% of obstetricians in the US had witnessed a maternal death attributable to β-agonist use [70].
β-agonists are diabetogenic and result in hyperglycemia due to gluconeogenesis and glycogenolysis stimulated by glucagon release from the pancreas. Insulin is produced to counteract this iatrogenic hyperglycemia and, in some diabetic women, insulin therapy may be required to treat ketoacidosis [71-74]. β-agonists also cause hypokalemia [75-77] following hyperglycemia, caused by insulin-induced potassium influx into the cells [78-80]. Hypokalemia causes arrhythmias, which increases the risk of pulmonary edema [51,76-78]. Rebound hyperkalemia after cessation of terbutaline [81] and ritodrine therapy [82] has been reported.
4.1.3.2 Fetal effects
β-agonists cross the placenta, enter the fetal circulation and may induce fetal tachycardia by directly stimulating the myocardium [48]. There is an increase in left ventricular stroke volume and cardiac output, a decreased pulsatility index of the umbilical artery and an increased pulsatility index of the middle cerebral artery [83]. The clinical significance of such changes in the fetal cardiovascular system is unclear and it is difficult to measure the extent of any myocardial ischemia in neonates [84]. β-agonists may induce hyperinsulinemia and hypoglycemia in the neonate, which may be reactive in response to maternal hyperglycemia [85].
4.2 Vasopressin/oxytocin receptor antagonists
4.2.1 Mechanism of action
Myometrial contraction depends on an increase in intracellular calcium. The action of oxytocin at oxytocin receptors causes a rise in intracellular calcium through a G protein-coupled mechanism involving secondary messengers such as IP3 (see Section 2). During term and SPTL, there is an increased expression of oxytocin receptors in the myometrium [86], as well as a significant increase in the amount of oxytocin secreted, with a higher frequency of pulsatile secretion [87]. In addition to this direct effect of oxytocin on the myometrium, PG production in the decidua is facilitated by oxytocin [86]. Atosiban is a synthetic competitive VOT receptor antagonist that binds to vasopressin (V1a) and oxytocin receptors in the myometrial cell membrane, resulting in i) dose-dependent inhibition of oxytocin-stimulated IP3 production and therefore a decreased release of calcium from intracellular stores in the sarcoplasmic reticulum of the myocyte; ii) closure of voltage-gated channels in myometrial cell membranes, preventing influx of calcium ions; and iii) prevention of oxytocin-mediated stimulation of PG secretion in the decidua and fetal membranes, further enhancing the negative effects in i) and ii) [88].
4.2.2 Efficacy
The first pilot study of atosiban was carried out on 13 patients in whom atosiban caused marked (in some cases total) inhibition of their uterine contractions, without any fetomaternal side effects [89]. This was followed by another pilot study that found inhibition of uterine contractions in nine out of 12 women treated with atosiban without any apparent side effects [90]. Goodwin et al. [91] published a triad of papers in which atosiban appeared to be effective in reducing myometrial contractility, with similar efficacy to ritodrine [92], while it was well tolerated by trial participants [93] and lacked the side effects of ritodrine [92].
The RCT by Romero et al. [94] was a Phase III placebo-controlled study of atosiban that allowed alternative tocolytic rescue if labor continued despite initial tocolytic therapy. Their primary outcome measure was the time from administration of atosiban or placebo to delivery or therapeutic failure. There was no significant difference in this end-point between the treatment and placebo arms of the trial, but the percentage of women remaining undelivered and not requiring tocolytic rescue with another tocolytic agent was significantly lower with atosiban at all three times of assessment (24 h, 48 h and 7 days). The effects of atosiban were more favorable in women who presented beyond 28 weeks of gestation.
The largest RCT of tocolytic therapy ever conducted involved atosiban [95]. This was a pooled analysis of three prespecified subgroup reports produced by collaborative groups from eight different countries [96-98]. This trial compared the effectiveness and safety of atosiban with that of three β-agonists (ritodrine, salbutamol and terbutaline). The primary end-point was the number of women remaining undelivered at 48 h and at 7 days. Using an intention-to-treat analysis, no significant difference in effectiveness was found between atosiban and β-agonist therapy. This analysis included women who did not receive study drug, those who were protocol violations, those who failed and had alternative tocolytic therapy and those in whom tocolytics were successful but who contracted again and had repeat courses. With this amalgam it is not surprising it was difficult to show a difference in effectiveness. When only those who received study drug as planned were compared, atosiban was found to have superior efficacy (a composite outcome of safety and tolerability) than β-agonists [95].
A double-blind RCT by Valenzuela et al. [99] compared the efficacy of atosiban maintenance therapy to placebo in women with SPTL who had achieved uterine quiescence withintravenousatosiban.Subcutaneousinfusionofatosiban significantly prolonged uterine quiescence (median of 32.6 days with atosiban versus 27.6 days with placebo) and treatment was well tolerated.
A controversial Cochrane systematic review was published in 2005 assessing the tocolytic effects of atosiban versus placebo or any other tocolytic agent [100]. This review included six trials involving a total of 1695 women and found no reduction in preterm birth with atosiban compared to placebo or β-agonists. Papatsonis et al. [100] also reported a decrease in infant birth-weight with atosiban treatment, as well as a higher frequency of maternal adverse drug reactions. However, the review has been criticized by a number of opinion leaders who have expressed concerns that there may have been unintentional bias in favor of CCBs and that the review’s methodology did not enable the outcomes to be evaluated. Others felt that there was no rationale behind the selection of the trials and that the review exemplified the drawbacksofmeta-analyseswithrespecttoselectionbiasand absence of quality weighting. One opinion leader, much of whose published research occupied the review, expressed his concerns that acknowledgement of his assistance implied that he concurred with their conclusion. He felt that the analysis was ‘flawed’ and ‘not up to the high standards of Cochrane Reviews’ [101]. Others recorded that the review contained a worrying degree of ‘subjectivism’ with respect to study inclusion and interpretation, which focused on data taken out of original context and without reference to subject profiles. A detailed critique of the shortcomings of the review and comments of opinion leaders is contained in a separate review [53].
4.2.3 Safety
4.2.3.1 Maternal effects
Atosiban has placebo-level fetomaternal and neonatal side effects or minor effects such as erythema around injection sites [99]. Atosiban was associated with only one-tenth of the cardiovascular side effects of β-agonists (81 versus 8%) and β-agonists resulted in a 15-fold increased risk (15 versus 1%) in the need to discontinue therapy due to unacceptable side effects [95].
Atosiban has affinity for vasopressin receptors (V1a, V1b and V2) as well as oxytocin receptors but no dangerous maternal complications pertaining to this have been reported to date, with no significant renal, cardiovascular or neurological complications. Theoretically, oxytocin antagonism in tissues that express oxytocin receptors may inhibit milk release or postpartum uterine contraction but the short duration and readily reversible effect of atosiban makes these risks negligible [102].
4.2.3.2 Fetal effects
Over the long history of atosiban use since the first pilot trials and during the worldwide randomized comparative trial [95] and clinical use, no substantiated significant safety concerns for the fetus have emerged. Atosiban therapy was associated with an increased incidence of perinatal death when compared to placebo in one study and this was largely responsible for the FDA denying
approval in the US in 1998 [94]. However, due to imbalances of randomization in that study, the atosiban treatment group had been allocated more women at a gestational age of < 26 weeks who were also significantly more advanced in SPTL (assessed by the modified Bishop score), so the treatment and placebo groups were unequal at baseline. Following approval in the European Union in 2000, as of June 2007, atosiban was approved in 67 countries, excluding the US and Japan. The calculated cumulative patient response to atosiban (January 2000 to December 2005) is estimated at 156,468 treatment cycles, which, with routine monitoring of drug safety, has revealed no important safety issues. Vasopressin is secreted by the fetus exposed to stress, as a homeostatic response [103] and it was thought that vasopressin antagonism by atosiban may have led to excess deaths in the treatment group compared to placebo. However, the authors explained that it was unlikely that atosiban had contributed to these deaths, as higher doses of atosiban had previously been associated with an improved perinatal outcome and experimental toxicity studies in animals had not revealed any adverse events. Fetal plasma atosiban levels have been shown to be negligible or undetectable [104].
4.3 Calcium channel blockers
4.3.1 Mechanism of action
Calcium channel blockers such as nifedipine and nicardipine block voltage-dependent calcium channels on the myometrial cell membrane. This blocks the influx of extracellular calcium into the smooth muscle cell. Since less intracellular calcium is available for binding to myosin light chain kinase and formation of the calcium–calmodulin complex, phosphorylation of the actin–myosin apparatus is inhibited [105].
4.3.2 Efficacy
No placebo-controlled trials have been performed to assess the efficacy of nifedipine tocolysis. The first pilot trial, which compared nifedipine to placebo, also compared nifedipine to ritodrine, with 20 women allocated to each group [106]. Nifedipine was found to have a promising effect on postponement of delivery but 90% of women treated with nifedipine suffered from flushing of the face, neck and chest and one woman had marked nausea. Subsequently, the majority of randomized trials have compared the tocolytic efficacy of nifedipine with that of ritodrine [107-113], while others have compared nifedipine to other drugs such as magnesium sulfate [114,115] or terbutaline [116,117]. The trials that compared nifedipine with ritodrine found no difference in the tocolytic effect but a decreased incidence of maternal side effects such as chest pain, nausea and vomiting in those treated with nifedipine [107-109,111-113]. These studies were of small scale and blinding was not possible due to the different routes of administration of the drugs. None of the nifedipine studies were reported in an intention-to-treat analysis.
Atosiban or nifedipine but not β-agonists are currently recommended for the treatment of SPTL on the basis of the poor side-effect profile associated with ritodrine therapy and the apparent similarity of efficacy shared by all three drugs [52]. Nifedipine remains unlicensed in the UK for use as a tocolytic agent. The guidelines advocating nifedipine use were based on results from two meta-analyses and a Cochrane systematic review [118-120]. The findings of these meta-analyses are summarized in Table 1. The quality of the studies included in the meta-analyses have been criticized for being of poor quality and suggestions were made that such reviews should not be used for making guidelines or recommendations [121].
4.3.3 Safety
4.3.3.1 Fetomaternal effects
The adverse fetomaternal effects of nifedipine have included maternal pulmonary edema [122,123], myocardial infarction [124,125], hypoxia [126] and atrial fibrillation [127]. Nifedipine is licensed for use in hypertension, due to its effect on vascular smooth muscle, causing vasodilatation [128]. This may lead to severe hypotension in pregnant women and has led to fetal distress, thereby necessitating emergency delivery by cesarean section in one case where nifedipine was used to treat pregnancy-induced hypertension [129] and death of a fetus after tocolytic therapy with nifedipine [130]. ‘Near misses’ where nifedipine tocolysis has resulted in severe maternal hypotension without lasting morbidity or mortality are probably under-reported [131]. Van Veen’s report [130] of fetal death associated with nifedipine tocolysis was followed by anecdotal evidence that cases of maternal hypotension severe enough to cause fetal compromise occur at a higher rate than suggested by the number of published case reports [132,133].
It is still uncertain whether nifedipine carries risks of teratogenicity. Although digital abnormalities have been observed in animals receiving high doses of nifedipine [134,135], nocongenitaldefectsinhumansasadirectresultofnifedipine exposure have been reported. The only long-term follow-up study of children who were exposed in utero to nifedipine tocolysis compared the behavioral–emotional outcomes, quality of life, motor function, parenting distress and childhood education of children exposed to nifedipine with those exposed to ritodrine in utero [136]. No significant differences in long-term outcome were observed. A long-term comparison to placebo would have been more meaningful and no such follow-up study has been performed to date.
4.4 Magnesium sulfate
The pharmacological mechanism of magnesium sulfate is not understood. It is thought to decrease myometrial contractility by competing with calcium for entry into the smooth muscle cell at voltage-gated calcium channels [137]. Modulation of calcium influx and competitive binding of magnesium with calcium storage sites in the sarcoplasmic reticulum of the
Table 1. Efficacy of nifedipine from three meta-analyses [118-120].
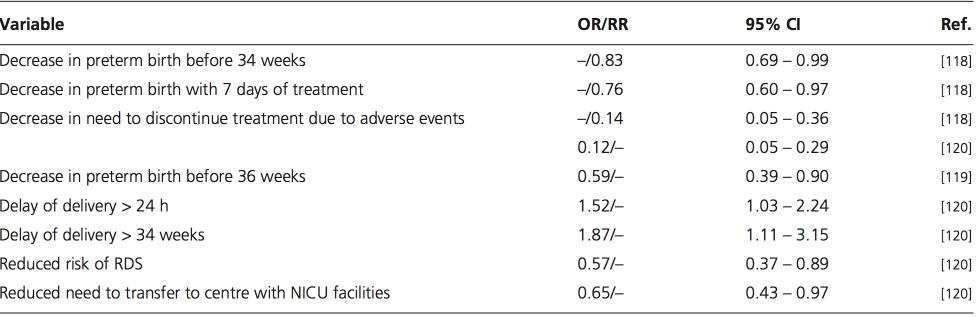
CI: Confidence intervals; OR: Odds ratio; NICU: Neonatal intensive care facilities; RDS: Respiratory distress syndrome; RR: Relative risk.
myocyte are possible mechanisms by which the frequency of smooth muscle depolarization can be decreased [48,138].
Magnesium sulfate is widely used in the US as a tocolytic agent. However, in two systematic reviews, magnesium sulfate was found to be ineffective as a tocolytic [57,138]. The current lack of evidence for tocolytic effectiveness of magnesium sulfate and the inadequacies in some studies have been reviewed in a recent commentary, which describes its continued use in North America as irrational and there have been calls for a ‘time to quit’ [139]. The risk of fetal and infant death was higher for babies exposed to magnesium sulfate (RR = 2.82 and 95% CI = 1.20 – 6.62) when seven trials involving 727 infants were analyzed [138]. This result was consistent with the Magnetic and Neurological End points Trial, which led to early suspension of the study [140]. Mortality in this trial was associated with higher doses of magnesium sulfate [141]. Antenatal exposure to higher levels of magnesium sulfate may also be associated with an increased risk of neonatal intraventricular hemorrhage [142]. The current evidence does not support the use of magnesium sulfate as a tocolytic.
4.5 Prostaglandin synthetase inhibitors
Prostaglandins, notably PGE2 and PGF2α, play a key role in the initiation and maintenance of labor [143]. Prostaglandin synthetase inhibitors such as indometacin or sulindac decrease myometrial contractility. Indometacin is the most widely studied PGSI used as a tocolytic in clinical practice since the early 1970s. Indometacin has been shown to result in a reduction in preterm birth and an increase in gestational age and birth-weight [144]. Two trials demonstrated a trend towards delay of delivery by 48 h and 7 days [145,146], but the sample size was small. There were also insufficient data to assess potentially adverse effects of PGSIs on the fetus, neonate or mother.
Fetal PGs have important roles in renal development, coagulation and vascular regulation. The ductus arteriosus is kept in its dilated state and the splanchnic and cerebral blood flows are both regulated by PGs [143]. Prostaglandin synthetase inhibitors cause transient and reversible effects such as reduction of flow in the ductus arteriosus and impairment of fetal renal function [147]. Other investigators have reported an increased risk of neonatal periventricular hemorrhage, premature closure of the ductus arteriosus, impaired renal function, necrotizing enterocolitis and bronchopulmonary dysplasia [148-150]. A meta-analysis assessing the neonatal safety of indometacin tocolysis concluded that the extent of these risks is unknown due to the limited statistical power of published RCTs [151].
4.6 Nitric oxide donors
4.6.1 Mechanism of action
The inducible form of nitric oxide synthase is present in rat endometrium and its expression decreases with the onset of labor [152,153]. Myometrial nitric oxide synthase expression decreases in a similar fashion during human parturition [154]. In the rat myometrium, contractility is increased by soluble guanylate cyclase or inhibition of nitric oxide formation, while it is decreased by L-arginine, the natural substrate for nitric oxide synthase [155,156]. The mechanism of action of nitric oxide inhibition of myometrial contractility is unknown.
4.6.2 Efficacy and fetomaternal effects
Very little evidence has been published on the tocolytic efficacy of nitric oxide donors. The only RCT of nitric oxide donor tocolysis with placebo controls suggested that glyceryl trinitrate (GTN), the only nitric oxide donor evaluated in clinical trials, does not significantly delay labor or improve neonatal outcome [157]. A later meta-analysis confirmed the result of this trial and reported that GTN therapy did not delay delivery when compared with other tocolytic agents over periods of 48 h or 14 days [158]. An RCT published after this meta-analysis found GTN to be less effective than β2-agonists as a tocolytic agent, especially when rescue tocolysis with β-agonists was regarded as treatment failure [159]. Another RCT comparing the tocolytic efficacy of transdermal GTN to that of intravenous ritodrine found no difference in prolongation of gestation to 37 weeks of gestation or in the proportion of women who delivered before prespecified time limits [160].
The action of nitric oxide on maternal vascular tone may lead to changes in uterine blood flow and fetal side effects. Vasodilatation may also lead to maternal side effects such as flushing, headache, hypotension and tachycardia [158]. The RCT by Lees et al. [160] found headache to be the commonest maternal adverse effect, but other side effects such as tachycardia, palpitations and nausea and were less common with GTN therapy than with ritodrine therapy.
5. Conclusion
Preterm birth is the major cause of perinatal mortality and morbidity in the developed world. Not all cases of SPTL are suitable for tocolytic intervention. The choice and use of tocolytics has evolved over the last 20 – 30 years. The perfect tocolytic, which is uniformly effective and has no fetomaternal side effects, does not exist. Only one tocolytic agent has been developed specifically to treat SPTL (atosiban) and this is the most utero-specific of all the agents. As a result, atosiban has placebo-level side effects while all other agents have multi-organ side effects, particularly cardiovascular adverse effects. Although more costly than other tocolytics, atosiban has the most robust evidence base for safety and efficacy of all available tocolytics and is a useful addition to the management of SPTL [95]. Since the remit of this review pertains to the pharmacotherapeutic management of SPTL, novel techniques of prediction and prevention are not covered. However, the reader is recommended to read the excellent review of the use of genomics, transcriptomics, proteomics and metabolomics for the prediction and diagnosis of SPTL [161].
6. Expert opinion
The incidence of preterm birth is underestimated [162] and is likely to increase due to increasing risk factors. The associated morbidity and mortality results in a huge burden of healthcare costs. Despite this there is a disarray and lack of consensus on the use of tocolytics [88], which must be due to confusion about the evidence supporting the efficacy and safety of each group of agents. The evidence supporting magnesium sulfate is poor and this should not be used as a tocolytic. The evidence supporting nitric oxide donors is scanty, their use is infrequent and they may cause substantive cervical changes that predispose to labor and they should not be used as tocolytics. While PGSIs have tocolytic effects, they have potentially serious fetal adverse effects and, since better alternatives are available, their use should be discontinued or extremely limited to third line management. β-agonists are licensed for use but are no more efficacious than CCBs or VOT receptor antagonists and have the potential to cause serious maternal adverse effects, particularly cardiovascular effects. Their use should be limited to second- or third-line choice. The main debate at present is between nifedipine and atosiban.
6.1 The case for nifedipine against atosiban
Those who favor nifedipine over atosiban will quote the systematic review [118] of CCBs (effectively nifedipine) versus β-agonists (effectively ritodrine) to claim that nifedipine is much more efficacious and safer than β-agonists. They will also quote the systematic review [100] of VOT receptor antagonists (effectively atosiban) versus β-agonists to claim that atosiban lacks safety and efficacy when compared to placebo or β-agonists. They will claim superiority of nifedipine over atosiban based on an indirect comparison of nifedipine versus atosiban [163] and the fact that nifedipine can be used orally and is inexpensive.
6.2 The case for atosiban against nifedipine
Those who favor atosiban over nifedipine will argue that most of the evidence pertaining to CCBs is contained in meta-analyses that are retrospective analyses of pooled data, which are only as good as the quality of the studies included. Studies included in the systematic review of CCBs versus β-agonists [118] have been criticized for being of poor quality, with small sample sizes, having no placebo-controlled trials or follow-up studies and for lacking robustness with respect to the requirements for regulatory bodies. They would also demonstrate that the evidence of randomization, concealment and attrition bias is such that these studies should not be used to make recommendations or guidelines [121,131]. This would be in contrast to the evidence base for atosiban, which is far more robust. In contrast to nifedipine, atosiban studies have included well-conducted, placebo-controlled, follow-up, prospective Phase I – IV studies as well as large multinational studies published on an intention-to-treat basis with no suggestion of bias. Those who favor atosiban will also criticize the systematic review of atosiban [100] and provide evidence for this being biased and flawed [53].
The comparisons between nifedipine and atosiban much quoted by nifedipine enthusiasts [162] were an indirect comparison using a mathematical tool that was self-critical of drawing any meaningful conclusion and two recent studies directly comparing nifedipine and atosiban that concluded that atosiban was as efficacious as nifedipine but had much fewer cardiovascular side effects [164,165]. In contrast to nifedipine, atosiban is licensed for use and is based upon large, well-conducted studies involving placebo-controlled trials and Phase I – IV and follow-up studies [166], was developed specifically to treat SPTL [88,102] and is utero-specific with placebo-level side effects. Following the decrease in β-agonist use, as the use of nifedipine has increased so have the recorded adverse events [122-127,130]. The cost will be justified based upon the research and development required to market a new utero-specific compound with placebo-level side effects developed specifically to treat SPTL, which meets the requirements to satisfy regulatory bodies, in comparison to the cost of preterm birth in general and in comparison to other drug budgets. Finally, those in favor of atosiban would counter their argument with respect to oral therapy by pointing out that rapid-onset preparations of nifedipine compromise safety, leading to the use of long-acting or slow- release preparations that compromise efficacy and lead to the use of additional tocolytics such as magnesium sulfate or β-agonists, which further compromise safety. The decision on whether to use nifedipine or atosiban should be based on the evidence available. There is no doubt that the evidence supporting the use of atosiban is more robust, more plentiful and of much higher quality. This evidence leads to the conclusion that atosiban is at least as effective as nifedipine and β-agonists with much fewer side effects than both, particularly cardiovascular ones and the need to discontinue therapy because of unacceptable side effects is decreased. Except for cost pressures, most units would prefer to use atosiban because they are convinced by the supporting evidence and because of the safety and lack of side effects.
For a number of reasons, pharmacotherapy for the management of SPTL may not be a high priority for the pharmaceutical industry. Firstly, the market is relatively small. Secondly, litigation costs are high. While the same worries of litigation could be said of diabetics, the huge market makes this condition more attractive for pharma- ceutical companies. Thirdly, two patients (mother and fetus) are involved in each treated case, which increases and complicates the data required to satisfy regulatory bodies. In this way, the cost of carrying out good quality studies to satisfy regulatory bodies may not be reflected in the reimbursement that governments and other healthcare providers are prepared to offer. A review by Goodwin [167] elegantly displays the hurdles that have to be overcome to satisfy the FDA. Two other oxytocin receptor antagonists are currently under evaluation and development: barusiban (Ferring, St Prex, Switzerland) and relcovaptan (Sanofi- Synthelabo Recherche, Paris, France). Relcovaptan (SR49059), like atosiban, is a VOT receptor antagonist with antagonistic activity against both the vasopressin V1a and oxytocin receptors in the uterus. In a randomized, double- blind, placebo-controlled trial of 18 women (12 of whom received relcovaptan and six placebo) in preterm labor between 32 and 36 weeks of gestation the frequency of uterine contractions over a 30-min period was significantly lower in the relcovaptan group compared to placebo-treated women [168].
In contrast to both atosiban and relcovaptan, barusiban (FE200440) has a high affinity for the oxytocin receptor, which is of the order of 300-fold that for the vasopressin V1a receptor. In animal studies using a model of oxytocin- induced preterm labor in non-human primates, barusiban was effective in suppressing such contractions with no long- term adverse events [169,170]. Whether such a selective oxytocin receptor antagonist would be as effective in spontaneous preterm labor (as opposed to oxytocin-induced preterm labor-like contractions) has not been demonstrated in human clinical studies and we await with interest the reports of a Phase II study of barusiban, which we understand is to be reported at the meeting of the Society for Gynecological Investigation in San Diego, US, in March 2008. Little is known about the use of combination tocolytic therapy. At a time when the cost and adverse sequelae of tocolytics are hot topics of debate, it seems counterintuitive to suggest using double or triple tocolytic therapy unless lower doses of each are used. In addition, if, due to infection, SPTL and preterm birth has ‘survival value’ for the mother or her fetus, three different tocolytics with different mecha- nisms of action may be powerful enough to combat the protective response of nature to empty the uterus rather than a single tocolytic, thereby causing more harm than good to the maternal host and the fetus. However, at the limits of viability combined tocolytic therapy (terbutaline, atosiban and sulindac) together with combined antibiotics (cephalexin and metronidazole) have been employed with surprisingly good results [171].
Declaration of interest
Ronald F Lamont has given lectures and advice and chaired sessions at conferences supported by Ferring Pharmaceuticals and sanofi-synthelabo.
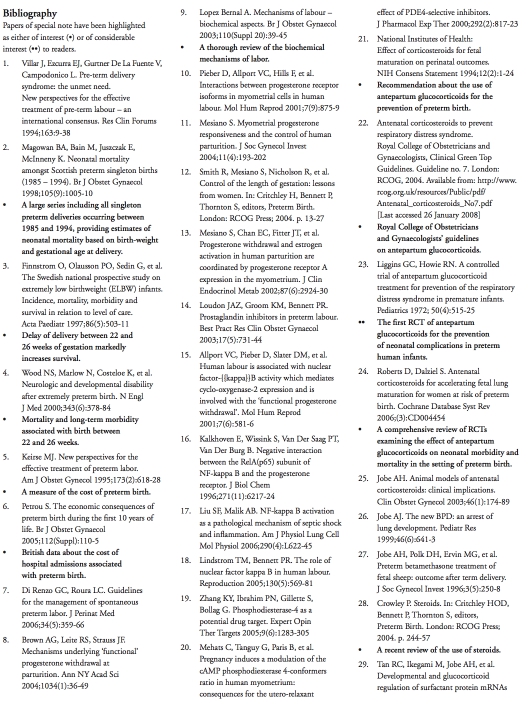
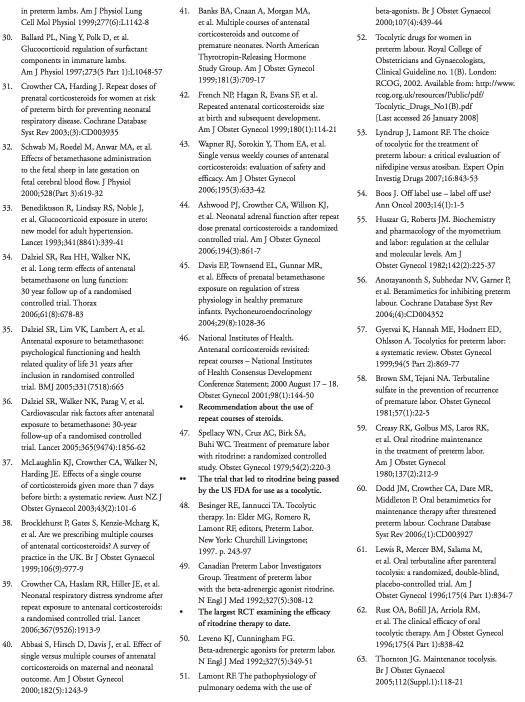
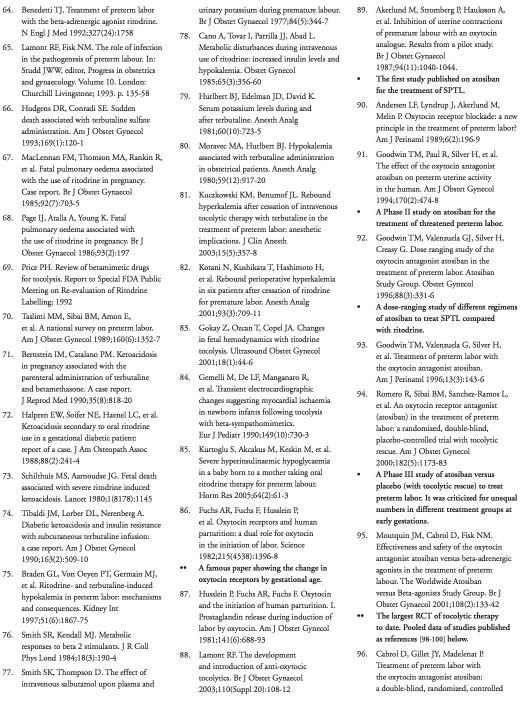
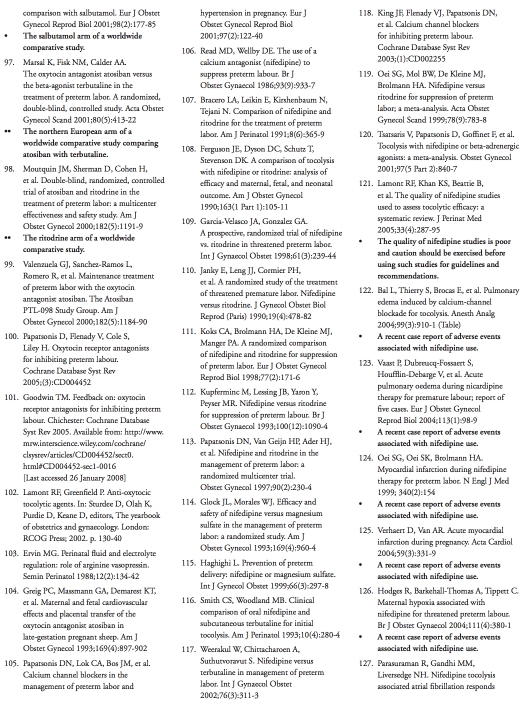
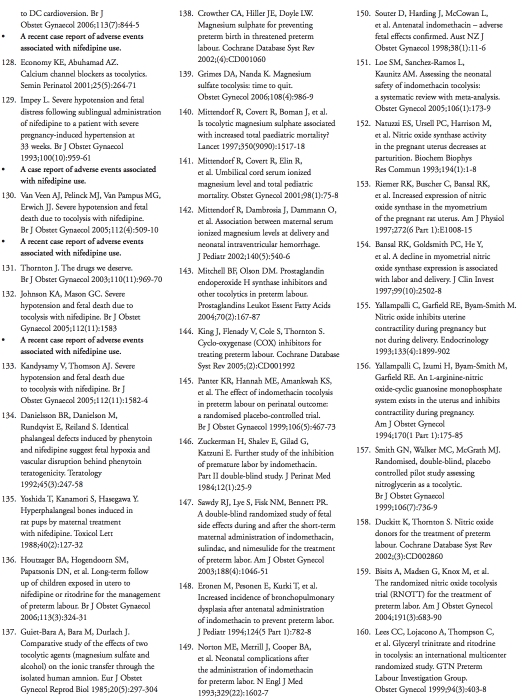
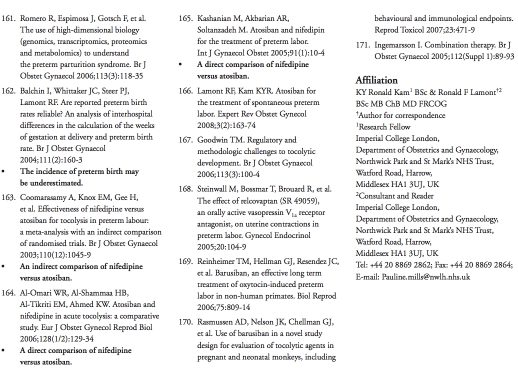